Anyone seeking information about essential oil quality soon learns about the existence of gas chromatography (GC) testing. Quite often GC and GC-MS (gas chromatography / mass spectrometry), are discussed as if they were synonymous. This is not the case, as GC and MS are two separate pieces of equipment, each with its own purpose.
Many in the aromatherapy community consider GC-MS to be virtually the only analytical method for essential oils. It is indeed the core of any valuable quality control procedure applied to essential oils, though there are in fact other analytical tools that can also be used, with variable relevance.
This post will cover some basics of GC and MS, and examine what GC-MS can… and cannot do. Above all, it should be remembered that GC-MS testing does not tell us everything.
First things first: “chromatography” in gas chromatography
Analytical chemistry’s goal, as a discipline, is to provide accurate compositional and quantitative data about the chemistry or related properties of a substance. It comprises relatively simple methods, such as titrations used to quantify, for example, the amount of acid in a dilute hydrochloric acid solution. This is a very simple case, because there are only two ingredients involved: water and hydrochloric acid. Yet, very often, the questions to be answered become more and more complex as the number of individual ingredients increases. At this point, if data are to be collected about one component in particular, the chemist has to separate it from the rest of the mixture to be able to study it.
Chromatography was born in 1900 when Mikhail Tsvet wanted to study pigments in plants, specifically chrorophylls. Tsvet gradually developed a technique to isolate individual chlorophylls from a plant extract. Since he was working on colored substances, he called his method “chromatography” (from the Greek khroma, meaning color). In just over a century, chromatography has evolved into several subtypes, each one allowing us to separate molecules for a plethora of pharmaceutical, environmental, chemical and biochemical applications. All these techniques use roughly the same basic concepts, which are discussed in this post.
The main purpose of any chromatographic process is to separate compounds so that we can then study them independently from the others. As essential oils are made of dozens, often hundreds of molecules, it is not surprising that gas chromatography is so fundamental in quality control.
Gas chromatography as a cornerstone of essential oil analysis
Let us now move to the famous GC in GC-MS: gas chromatography. It is a specific branch of chromatography that does what it has to do in general very well – that is, separate a large number of molecules in a reasonable timeframe. Gas chromatography is limited by the fact that, as its name suggests, its operation relies on gases, and so the molecules being separated must be gaseous. In plants, most compounds, for example sugars and pigments, are not volatile. Their boiling point is so high that they usually degrade due to high temperatures before becoming gases. But essential oils are, by nature, composed of molecules that are volatile, and so readily shift to a gas phase. This type of chromatography is thus the most logical choice to study essential oils. A more detailed discussion about the principles of gas chromatography can be found here. What has to be kept in mind is that on its own, a GC instrument does only that: separate molecules and then release them. It gives no information on what they are, and how much of each compound is present. This is where the detector comes into play.
The mass spectrometer – and friends!
If there is nothing at the end of a GC instrument, the compounds are simply released from the chromatographic column into the air, separated from each other. This is all but useless, because the objective of this separation was to get some information about these molecules individually. Usually, we want to know what are these molecules, and how much of each is present.
This is why virtually no one ever performs strictly a GC separation. The GC separates, and then the detector allows for analysis of the data. GC-MS (in the long form, gas chromatography coupled to mass spectrometry) is only one of several possibilities: GC-FID (flame ionization detector) is also very common, and other detectors include TCD (thermal conductivity detector), ECD (electron capture detector), FPD (flame photometric detector), IRD (infrared detector) and so on. Some exotic detectors include the human nose for GC-olfactometry/GC-sniff (useful to study the aromatic details of wines and perfumes for example, so why not! ) and insect antennas in electroantennography (to study the effect of volatiles on insects’ nervous system) .
So MS is only one of several possibilities for the analysis of compounds in GC. I am stressing this because, in North America, it is often wrongly presented as being almost the only valid instrumentation for essential oils analysis.
How does a mass spectrometer (MS) work?
Although a more detailed explanation can be seen here, it can be briefly described as follows. Upon entering the detector, molecules are broken down into fragments, which are then individually sorted according to their mass, and counted. The whole process is very quick, and repeated several times per second.
Now let’s look at what GC-MS can and cannot do.
– GC-MS can identify a large number of volatile molecules
Depending on their structure, molecules will yield different fragments. And it is important to remember that one compound reaching the detector is in fact millions of the same molecule entering the detector at once. Some fragments are easier to produce than others for a given molecular structure, and will statistically occur more frequently than others. The end result is a mass spectrum (Figure 2) that recaps the proportions of all mass fragments observed at one point in time by the detector. This spectrum can then be compared to databases of reference compounds. The same molecule, treated with comparable MS instruments, will yield very similar mass spectra, which can be considered as a fingerprint.
MS is very efficient for carrying out identity checks. Very often, the fingerprint will be quite specific for one molecule. Over time, large databases of reference compounds have been assembled, such as the National Institute of Standards and Technology database (NIST14, currently, is sold with many GC-MS instruments) and the Wiley Registry, as well as some specialty libraries like the seminal work of Robert P. Adams on essential oils or the library of Luigi Mondello, an Italian reference in the same field. Many laboratories also build their own databases over time, either from standards or by comparison with scientific literature, which also contains many spectral references.
So, in a screening approach, MS is a powerful tool to identify compounds.
– But MS cannot identify all molecules
GC-MS is in general an excellent technique to provide identity, but there are some exceptions to this. First, a MS search produces a list of hits, some of which are incorrect. For example, citronellyl acetate will often match with 2,6-dimethyl-2,6-octadiene, and linalool with linalyl anthranilate among possible identifications, and there are trickier instances, especially for rarer compounds. In some cases, different compounds will yield quite similar mass spectra. This is the case of several cyclic cis/trans isomers and some sesquiterpenes . In those cases, the use of the mass spectrum alone is not sufficient, and one has to include the retention index technique. This can be easily implemented in GC-MS, and solves this challenge.
Furthermore, plants are very complex from a chemical point of view, and essential oils are no different. Many oils, including some common ones, feature compounds that are simply unknown to science; vetiver is a good example of this . If no one had ever isolated a specific molecule before, then of course, the mass spectrum for that molecule would not find a match in the database. Or, it might match with the wrong candidate, leading to a misidentification. The only way out in such cases is to isolate and fully characterize the molecule, which is labor-intensive. Still, the mass spectrum can give us some information about a molecule. For example, the analyst can formulate a hypotheses pertaining to the class (sesquiterpene, phenylpropanoid…) of the compound, and sometimes deduce the number of carbons, hydrogens, and oxygens present in the structure.
– MS can give an indication of compounds’ proportions
In chromatography, most of the time, quantitation is based on a calibration built for each target molecule. For example, if you want to know exactly how much eugenol there is in a clove oil, you can prepare solutions containing various concentrations of pure eugenol and compare those with the clove sample by injecting all of them using the exact same method on a GC instrument. This approach, regardless of the detection method, is the most reliable to get an accurate quantitation of a compound, and can be applied in MS using a special mode called single-ion monitoring (SIM) .
In essential oils, the calibration task is much more daunting. There are so many molecules at once that it is highly impractical to build a calibration for each of them . Furthermore, many of these molecules are not available as pure standards. The most widely applied approach chosen to circumvent these obstacles is called internal normalisation . It consists of measuring the signal (the electronic output of the detector) corresponding to each peak in the sample, and considering that the ratio of this signal compared to the sum of all signals detected equals the percentage of the associated molecule in the matrix. This is where the percentage format comes from. A eugenol peak representing 35% of the total signal of the injection will be considered as representing 35% of the sample. This is a practical, commonly accepted approximation, but an approximation nonetheless (more about this can be found here and here). Several experts, as well as the ISO norm (see box below), suggest instead using a FID detector for relative compounds quantitation, with MS serving as a side detector to confirm compound identities .
The International Organization for Standardization issues norms applied to a great variety of fields, with the objective of achieving greater reproducibility, compatibility and standardization among institutions and businesses around the world. Several of these standards have been prepared in the field of essential oils, and describe expected oil compositions and relevant methods applied for testing.
This is why some GC-MS equipment has a built-in FID detector. At the end of the column, compounds are split into two tubes, each leading to one of the detectors. The signal from the MS is applied to identification, whereas the signal from the FID is applied to relative quantitation.
All in all, MS can still be used to get a good idea of the relative concentration of compounds in an essential oil, but it is not the ideal detector for this task.
– GC-MS helps confirm botanical source
Since this kind of testing allows us to identify a large number of volatile constituents, GC-MS can be used to detail constituents of an essential oil, with their respective proportions. This in turn can be used to confirm a stated botanical origin (for example, to discriminate between lavender and lavandin oils), determine chemotypes (e. g. linalool vs methylchavicol basil), and even confirm ingredients from an oil blend. In some cases, the geographic origin of samples can also be confirmed (e. g. Haitian vs Java vetivers, the two types being different). These techniques are based on knowledge of plant-specific markers, and essential oils’ expected variations.
– GC-MS is not ideal for comparing ISO/AFNOR norms
Several norms for essential oils have been developed by the ISO organization, as well as the French governmental body of normalization, the AFNOR. Some other standards exist, such as those developed in Australia for local species. In most cases, these norms were developed statistically from data acquired with GC-FID . For various reasons, these cannot be considered equivalent to GC-MS. As such, using only MS-based quantitation to attest ISO/AFNOR compliance is not ideal.
– GC-MS can be used to compare similar essential oils
Let us imagine that a farmer would like to select the best cultivar of rosemary for his oil production. GC analyses in general (including GC-MS) can help comparing the results obtained for each cultivar to reach, for example, a given content of verbenone. Similarly, systematic GC testing could be used to optimize cultivation parameters. Other applications would include screening wild plants from a given area to check for possible chemotypes, and assess variation of the population.
However, as indicated above, MS is not the best choice for relative quantitation. If the oils to be compared vary significantly in compounds’ identity or proportions, it is recommended to also use FID detection to establish statistics, and even further, to apply some correction factors .
– But not so much to compare laboratories
MS detectors are relatively complex compared to several other detectors. This means that there are more parameters to set up, and that the results will be influenced by the way the instrument is used (especially in regard to the actual amount of sample that is injected). Furthermore, the instrument has to be frequently calibrated. This means that, unless special care is taken to reproduce these parameters and the same calibration, chances are that some results will be skewed in some way, especially for the fact that MS detectors saturate more quickly than other types of detector. In the case of essential oils, this makes it difficult to readily compare results from various laboratories. Lab-to-lab reproducibility is easier to achieve with other detectors, such as FID or TCD, that feature a simpler design and are thus more easily standardised in their operation.
– GC-MS is of considerable help in detecting adulteration and contamination
Although there are cases in which this method alone will prove insufficient to assess adulteration, GC-MS is of great help to identify compounds that should not be found in a given oil . Common examples include dihydrolinalool in linalool-rich oils, which is a marker of synthetic linalool addition. Similarly, the MS can be used to spot contaminations that would arise from accidental contact between two oils (by identifying markers of another oil in a given sample) or small amounts of solvents, such as phthalates or hexanes. The addition of artificial fragrance compounds is also best detected using GC-MS, because the range of possible compounds escalates, and a mass spectral confirmation of identification becomes crucial.

Figure 3: 4-Hydroxyisophthalic acid (4) is a subproduct of salicylic acid synthesis from phenol . During methylation to produce methyl salicylate it also produces dimethyl 4-hydroxyisophthalate (5) which is used as a marker.
– GC-MS may detect furanocoumarins
Citrus oils, as well as oils from some Apiaceae, often contain phototoxic furanocoumarins. Using a sufficiently heated method (since furanocoumarins are semi-volatile compounds, eluted much later than most other constituents of essential oils), their presence can be assessed with GC-MS. If a precise quantitation is required, however, a fully calibrated determination is required. This can be done using a variety of techniques, including GC-MS, but it is often recommended to go for liquid-based chromatography in those cases .
– GC-MS gives partial indications of oxidation
Essential oils, especially monoterpenes-, citral- and linalool-rich oils, are sensitive to oxidation. The result of oxidation varies depending on the compounds that undergo oxidation. Monoterpenes such as limonene and pinenes will first form peroxides, which are partially unstable in GC but are sometimes detected when oxidation is very severe . These peroxides eventually break down into other compounds, mostly alcohols , which can also be traced in GC-MS to check for oxidation of old orange oils for example. Aldehydes will rather be oxidized to related acids, which are readily spotted in GC-MS. This can help to check ageing of, for example, litsea, lemongrass or melissa oils. Other unusual compounds, such as di-alcohols, will also slowly arise from oxidation, and can be detected in GC-MS, although many of these are poorly reported in databases.
Assessing the full oxidative state of an essential oil can be a complex task. A recent review on the subject suggests using peroxide determination (more information here) and a method based on pH/conductivity measurement of water that has been briefly put in contact with an essential oil so as to extract oxygenated molecules to track oxidation.
– GC-MS can be used to check for rectification
Rectification involves redistilling or fractionating an oil in some way to correct some problems. This allows, for example, to increase the content of 1,8-cineole in eucalyptus, or remove the furanocoumarins from bergamot. In all cases, this has an impact of observed composition compared to a “crude” oil. Depending on the compounds present and their amount, the GC-MS data readily indicates if such rectification has taken place, as long as reference data is available.
– GC-MS usually distinguishes between distilled oils and other products
Absolutes, resinoids, oleoresins, expressed oils, solvent extracts and CO2 extracts are all possible in the world of aromas and fragrances. In almost all cases, the composition of these other plant-derived fragrant extracts will differ from that of the distilled oil. The analysis of composition provided by GC-MS can usually tell this difference. A good example is ginger distilled oil vs ginger CO2 extract. Several constituents will be similar early on in the chromatogram, but upon longer analysis, the CO2 extract will be shown to contain a set of compounds that cannot be steam-extracted, related to the gingerol series (Figure 5). Another example would be detection coumarins and flavonoids in expressed citrus oils, which are not carried over by distillation.
Color, texture and odor can also be of use to distinguish products of these extraction methods.
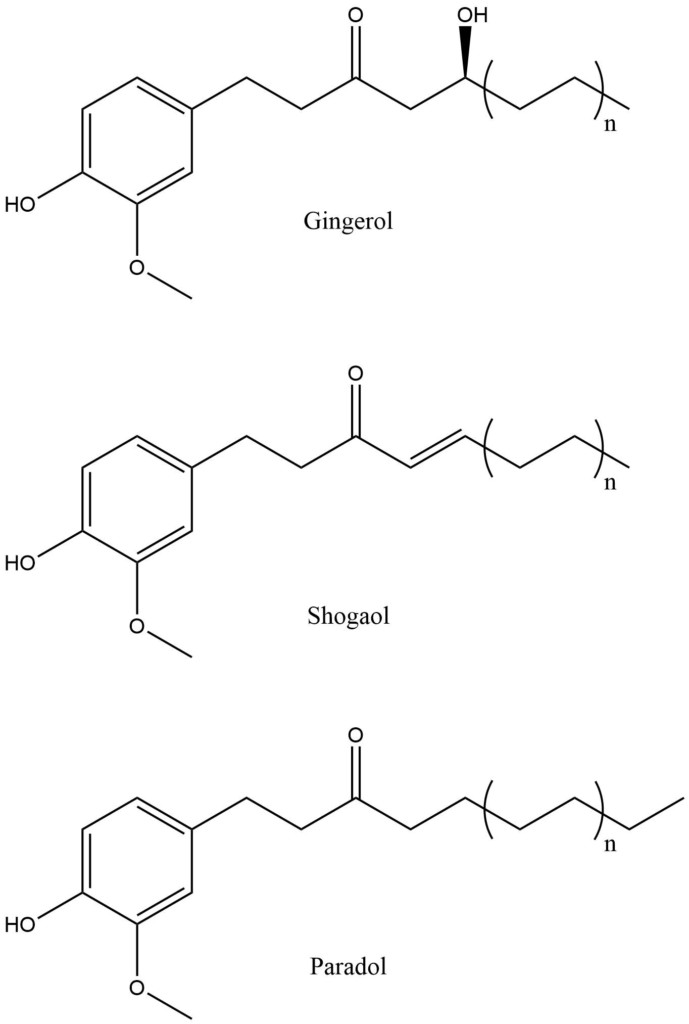
Figure 5: Gingerols series, n is a pair integer: 1, 2, … through 6. Each of them is named according to the length of the side chain, the most common one is -Gingerol with n=2. These compounds give the spicy taste of ginger and are not found in the essential oil.
– Classical GC-MS cannot find pesticides, but some variants can
Pesticides in general are found at very low levels in essential oils. Most GC-FID or GC-MS techniques used to establish the composition of an oil will stop at most at 0.01-0.05% of sample. In practice, compounds accounting for more than this proportion of the sample can still be neglected, due to coelutions or electronic noise in the detector. Either way, pesticides are present at the ppm (0.0001%) or even ppb (0.0000001%) level. In its most classical application in the field of essential oils, thus, GC-MS (or GC-FID, or GC-TCD) is unable to achieve the sensitivity required to check for pesticides. Some targeted applications of GC-MS looking only for specific ions, or ECD, a radioactive detector which is very sensitive to molecules bearing an halogen (chlorine, bromine, etc.), or specialized versions of mass spectrometers called tandem mass spectrometry (MS/MS) are more often used to achieve pesticides testing, and this is beyond the scope of the current discussion.
In general, pesticide analysis is a highly targeted task, requiring the analyst to look for pesticides and nothing else with usually costlier equipment and dozens of calibrations. So a pesticide test will tell you only about pesticides, and not about the rest of the oil’s composition. The general oil-oriented GC-MS testing will thus be useless to tell between organic and non-organic oils. Also keep in mind even organic labels do not warrant absence of pesticides – it just means that the farmer has not used them willingly, but tells nothing about his neighbours…
– GC analyses in general cannot detect heavy metals
Heavy metals, as well as most of their derivatives (save for a few exceptions) are not volatile enough to be caught by GC-MS. Furthermore, their level is, just as for pesticides, much lower than the usually applied limit of quantitation achieved with standard GC testing for essential oils. Assessing heavy metals require entirely different techniques, inspired by environmental analytical chemistry. Nowadays, this is usually done using induced coupled plasma (ICP) along with a mass detector, a technique that has little to do with GC-MS except for the fundamental way a mass detector works.
– GC-MS does not give information on non-volatiles
Gas chromatography (regardless of the detector) is useless to study non-volatile molecules. The most commonly used column in GC tolerates temperatures up to 325 °C. Beyond that point, the column itself degrades. So, any compound that does not reach a gaseous state in a stable form below this point within the conditions used in a GC injection is GC-proof. There are some solutions to this, but in a screening perspective (such as in essential oils, where we want to get a global fingerprint of the composition), these molecules will not be seen. The very way essential oils are produced make them perfect candidates for gas chromatography, as non-volatiles are left behind during distillation. However, absolutes, resinoids and CO2 extracts, as well as crude resins such as Peru balsam or copaiba, might contain non-volatile molecules that simply do not enter the column.
– GC-MS cannot tell you if a given compound is natural or synthetic
First, please do take the time to consider what, in the end, is natural or synthetic.
Not all adulterants can be spotted using markers. If no residual impurity from synthesis subsists in the added ingredient, other, more specific tests than GC-MS might be required to find it. Petroleum-based molecules can be found using carbon 14 testing. Many compounds are created from transformations of other natural building blocks, such as pinenes. Depending on the situations, checking for some isotopic ratios between carbon 13 and carbon 12, or deuterium and hydrogen, can reveal the culprit . For some molecules like linalool, the synthetic product often is a mixture of two versions of linalool (called enantiomers) that are not distinguished using regular GC-MS, but can be using specialized chiral GC: plants often produce specific ratios of enantiomers that synthesis may not be able to reproduce .
But there are cases where even all of those tests would probably not tell you about the occult addition of a compound, and you would need to get back to using several ratios between constituents of the oil to try to find adulteration. In most cases, these tests require quite extensive knowledge of what is usual and what is not. With the rapid evolution of essential oil-bearing plant cultivation worldwide, it is hard to keep track of reliable data that both excludes adulterated material, and does not penalize new producers that simply have to deal with natural variations within species due to local conditions.
– GC-MS does not give complementary information about essential oils
Gas chromatography (regardless of the detector) leaves aside several other aspects of quality control applied to essential oils. Good old physical measurements such as refractive index, specific gravity/density, or optical rotation, are still of value. And of course, an organoleptic analysis is always a good starting point to tell whether an oil suits your needs or not.
Summary
- GC-MS consists of two distinct parts, the gas chromatograph which separates constituents, and the mass spectrometer which is one of several types of detectors;
- It is an excellent tool to identify volatile molecules;
- It is a possible tool to quantify relative amounts of each constituent in an essential oil, although not the best in the case of essential oils;
- GS-MS is a cornerstone of essential oil analysis, that has however some limitations, especially with semi-volatiles and unknowns;
- It is a method that tells a lot, but not everything, and has to be complemented with specialized methods to answer specific questions such as oxidation level, adulteration with nature-identical compounds, or the presence of heavy metals for example.
I hope this discussion has given you a deeper understanding of what this technique is all about, including some of its possibilities and limitations.
References
Brattoli, M., Cisternino, E., Dambruoso, P. R. et al (2013). Gas chromatography analysis with olfactometric detection (GC-O) as a useful methodology for chemical characterization of odorous compounds. Sensors, 13(12), 16759–16800. https://www.ncbi.nlm.nih.gov/pmc/articles/PMC3892869/pdf/sensors-13-16759.pdf
Moorhouse, J. E., Yeadon, R., Beevor, P. S., Nesbitt, B. F. (1969). Method for use in studies of insect chemical communication. Nature, 223, 1174-1175. DOI: 10.1038/2231174a0
Adams, R.P. (2007). Identification of essential oil components by gas chromatography / mass spectrometry, 4th ed., Allured Publishing Corporation, Carol Stream.
Joulain, D., König, W. A. (1998). The atlas of spectral data of sesquiterpene hydrocarbons, E.B.-Verlag, Hamburg.
Belhassen, E., Filippi, J.-J., Brévard, H. et al (2015). Volatile constituents of vetiver: a review. Flavour & Fragrance Journal, 30(1), 26-82. DOI: 10.1002/ffj.3227
Bicchi, C., Liberto, E., Matteodo, M. et al (2008). Quantitative analysis of essential oils: a complex task. Flavour & Fragrance Journal., 23(6), 382-391. DOI: 10.1002/ffj.1905
Essential oils – Analysis by gas chromatography on capillary columns – general method, ISO 7609:1985.
General guidance on chromatographic profiles – Part 2: Utilization of chromatographic profiles of samples of essential oils, ISO 11024-2:1999(F).
Do, T. K. T, Hadji-Minaglou, F., Antoniotti, S., Fernandez, X. (2015). Authenticity of essential oils. TrAC Trends in Analytical Chemistry, 66, 146-157. DOI: 10.1016/j.trac.2014.10.007
Oils of bergamot, lemon, citron and lime, fully or partially reduced in bergapten – Determination of bergapten content by high-pressure liquid chromatography (HPLC), ISO 7358:2003.
Schieberle, P., Grosch, W. (1989). Potent odorants resulting from the peroxidation of lemon oil. Zeitschrift fuer Lebensmittle-Untersuchung und – Forschung, 189(1), 26-31. DOI: 10.1007/BF01120443
Chiron, F., Chalchat, J. C., Garry, R. P. et al (1997). Photochemical hydroperoxidation of terpenes I. Synthesis and characterization of α-pinene, β-pinene and limonene hydroperoxides. Journal of Photochemistry & Photobiology A: Chemistry, 111, 75-86. DOI: 10.1016/S1010-6030(97)00184-6
Turek, C., Stintzing, F. C. (2013) Stability of essential oils: a review. Comprehensive Reviews in Food Science and Food Safety, 12, 40-53. DOI: 10.1111/1541-4337.12006
Mosandl, A. (1995) Enantionselective capillary gas chromatography and stable isotope ratio mass spectrometry in the authenticity control of flavors and essential oils. Food Reviews International, 11(4), 597-664. DOI: 10.1080/87559129509541063
Can you please let me know if the most volatile components of oils can be measured by GC/MS or do they boil off too quickly?
Very great article! Enjoyed it to bits! I am also involved in essential analysis USING GCMS. I need more references ON USING RETENTION INDICES TO SUPPORT ms LIBRARY INFORMATION ESPECIALLY FOR ISOMERS AND UNKNOWNS.
Hello Mr. Murigi,
Good references about the usage of retention indexes include the famous book of Robert P. Adams: https://www.researchgate.net/publication/283650275_Identification_of_Essential_Oil_Components_by_Gas_ChromatographyQuadrupole_Mass_Spectroscopy
You can also refer to this review as a starting point: https://onlinelibrary.wiley.com/doi/pdf/10.1002/ffj.1887
Very useful